The epigenetic clock is a biomarker of aging
Epigenetic alterations, which are one of the nine hallmarks of aging, have been widely studied over the past ten years. Their in-depth study has led to an understanding of age-related changes affecting different epigenetic mechanisms, namely DNA methylation, histone modifications and chromatin remodelling. DNA methylation is a process in which methyl groups (CH3) graft onto specific DNA sites, thus condensing chromatin and preventing gene expression. The specific sites targeted by methylation are cytosines (one of the four DNA bases) belonging to CpG dinucleotides (a cytosine followed by guanine in the DNA nucleotide chain). These methylations occur naturally and allow the regulation of gene expression. However, with age, methylation alterations may occur, either as hypermethylation or hypomethylation. These changes in the methylation state of a gene can be measured, thus constituting biological markers of aging. The variation of these epigenetic phenomena during aging is called the epigenetic clock and allows us to evaluate our biological age[1], which often differs from our chronological age. Measuring biological age would make it possible to measure aging much more accurately, and opens up new therapeutic avenues against aging.
![In his latest publication[1], Steve Horvath refers to his epigenetic clock as the most powerful currently available. In addition to estimating chronological age with great accuracy, Horvath's epigenetic clock is also the only one that can be applied to different tissues. Indeed, it is the first to accurately measure the epigenetic age of our cells, tissues and/or organs in a distinct and specific way[1,3]. This specificity is one of the most important characteristics of Horvath's epigenetic clock, and makes it the most widely used estimator in anti-aging research[1]. In addition to this, the Horvath clock offers a panel of 353 CpG sites whose methylation profiles have been identified and classified: 193 hypomethylated CpG sites and 160 hypomethylated CpG sites with age. Epigenetic clock and age-related diseases](https://www.longlonglife.org/wp-content/uploads/2018/10/epigenetic-clock-long-long-life-aging-longevity-transhumanism-genetics-dna.jpg)
Today, the metrology of aging is a growing science with increasingly reliable biomarkers. The epigenetic clock has been reported to be the most promising biomarker for estimating biological age[2]. In the fight against aging, studying biological age differences between individuals of the same chronological age could help to understand the influence of internal and environmental stressors on aging[1]. Developing this powerful marker will help to understand, slow down, stop or reverse aging[1].
Epigenetic clock – how does it work?
The epigenetic clock is based on changes in the DNA methylation profile. There are 28 million CpG dinucleotides in the human genome[3], several million of which have an altered methylation profile with age. Several sets of tens or even hundreds of these CpG sites have been built and studied to create a biological age estimator: the epigenetic clock.
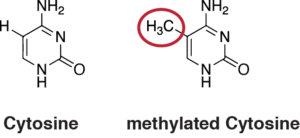
The epigenetic clock determines the biological age of our cells, tissues and organs. The age estimated by the epigenetic clock is also called the epigenetic age or “DNAm Age”. The epigenetic clock also gives rise to another concept: age acceleration, a measure corresponding to the difference between the age predicted (biological age) by the epigenetic clock and the chronological age. A German study conducted on a large population of elderly people associated the development of cancers and an increase in mortality due to cardiovascular disease with the acceleration of aging[4].
The different epigenetic clocks
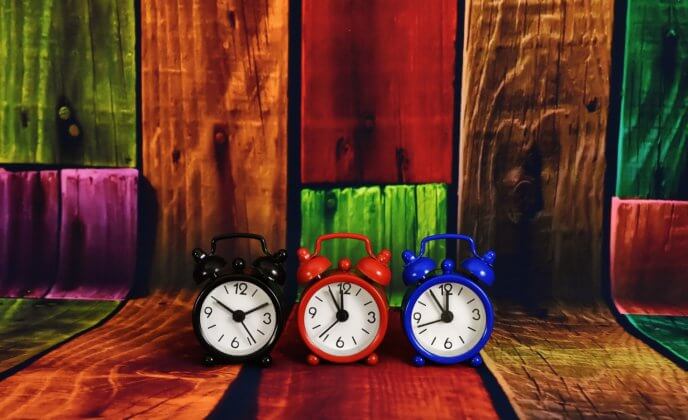
There are currently three epigenetic clock models, namely the clocks of Hannum, Weidner and Horvath. These clocks differ mainly in the age-related CpG sites used to build these biomarkers. Indeed, James Horvath uses a set of 353 CpG sites for his clock, while Gregory Hannum uses a set of 71 sites, and Carola Ingrid Weidner uses 3[5]. It should be noted that in order to estimate the chronological age as accurately as possible, it is preferable to have a large set of CpG dinucleotides. The larger the sets, the more accurate and robust the estimators are. Horvath and Hannum clocks are therefore currently the best estimators[2]. These two epigenetic clocks show a high correlation between predicted age and chronological age, with an average difference of 3.6 years for Horvath’s and 4.9 years for Hannum’s[2].Horvath and Hannum’s epigenetic clocks are also able to predict mortality independently of conventional risk factors such as age, weight, smoking and alcoholism, among others[2], based solely on the methylation profiles of CpG sites associated with age.
In his latest publication[1], Steve Horvath refers to his epigenetic clock as the most powerful currently available. In addition to estimating chronological age with great accuracy, Horvath’s epigenetic clock is also the only one that can be applied to different tissues. Indeed, it is the first to accurately measure the epigenetic age of our cells, tissues and/or organs in a distinct and specific way[1,3]. This specificity is one of the most important characteristics of Horvath’s epigenetic clock, and makes it the most widely used estimator in anti-aging research[1]. In addition to this, the Horvath clock offers a panel of 353 CpG sites whose methylation profiles have been identified and classified: 193 hypomethylated CpG sites and 160 hypomethylated CpG sites with age.
Epigenetic clock and age-related diseases
All aging diseases are caused by a cellular disorder that occurs at some time in our lives. These disorders tend to accelerate with age and increase our biological age, which will differ more and more from our chronological age: it is the acceleration of aging. This process reflects premature tissue aging and is associated with many age-related diseases. Indeed, the acceleration of aging is associated with a high risk of developing certain cancers and mortality related to these cancers[6]. In the same way, all cancerous tissues show signs of accelerated aging[3]. Accelerated aging is also associated with diseases such as cardiovascular disease[6], Parkinson’s disease and Werner’s syndrome, a disease that shows an accelerated aging phenotype. Finally, Down syndrome is associated with an acceleration of epigenetic age in the blood and brain[3]. Since the epigenetic clock applies to many tissues, it makes it possible to compare biological age differences between the organs of the same individual, and thus to detect a tissue that shows an acceleration of aging, implying the development of a pathology[3].
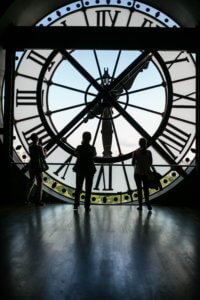
The epigenetic clock is a biological age estimator and marker of aging. Its robustness and its application to different DNA sources make it the best estimator currently available. The age predicted by DNA methylation allows us to study human development, aging and age-related pathologies. It can also become a good tool for evaluating anti-aging therapies[3]. In the longer term, the aim of this biomarker of aging will be to identify and validate epigenetic anti-aging interventions on humans.
References :
[1] Steve Horvath and Kenneth Raj. DNA methylation-based biomarkers and the epigenetic clock theory of ageing. Nature Reviews | Genetics (April 2018)
[2] Jylhävä J., Pedersen N. L. & Hägg S. Biological age predictors. EBioMedicine 21, 29–36 (2017).
[3] Horvath Genome Biology, 14:R115 http://genomebiology.com//14/10/R115
[4] Perna et al. Clinical Epigenetics (2016) 8:64 DOI 10.1186/s13148-016-0228-z
[5] Weidner et al. Genome Biology 2014, 15:R24 http://genomebiology.com/2014/15/2/R24
[6] Declerck, K., Mechanisms of Ageing and Development (2018), https://doi.org/10.1016/j.mad.2018.01.002
Anne Fischer
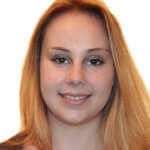
Author
Auteur
Anne is studying medicine science at the Institute of Pharmaceutical and Biological Science in Lyon and she has graduated with a Bachelor’s degree in molecular and cellular biology at the University of Strasbourg.
More about the Long Long Life team
Anne étudie les sciences du médicament à l’Institut des Sciences Pharmaceutiques et Biologiques de Lyon. Elle est titulaire d’une licence en biologie moléculaire et cellulaire de l’Université de Strasbourg.
En savoir plus sur l’équipe de Long Long Life
Dr Guilhem Velvé Casquillas
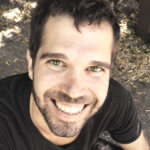
Author/Reviewer
Auteur/Relecteur
Physics PhD, CEO NBIC Valley, CEO Long Long Life, CEO Elvesys Microfluidic Innovation Center
More about the Long Long Life team
Docteur en physique, CEO NBIC Valley, CEO Long Long Life, CEO Elvesys Microfluidic Innovation Center
En savoir plus sur l’équipe de Long Long Life